Your cart is currently empty!
The Science of Satellite Communication
Back to: Communications Satellites Connecting the World
Overview
This lesson provides a comprehensive exploration of the scientific principles underlying satellite communication. By examining the physics of signal transmission, orbital mechanics, and the intricate roles of satellite components, learners will uncover how satellites facilitate global connectivity. The lesson also addresses the complexities of maintaining effective communication through the vastness of space and the challenges inherent to this process. Learners will not only understand the foundational concepts but also appreciate the cutting-edge technologies that make satellite communication a reality.
Learning Objectives
By the end of this lesson, participants will:
- Identify and evaluate challenges such as signal interference, latency, and orbital debris.
- Understand the fundamental principles of electromagnetic wave transmission and their critical role in satellite communication.
- Explore orbital mechanics and the importance of precise satellite positioning.
- Analyze how satellite components, such as transponders and antennas, contribute to the functionality of communication systems.
The Fundamentals of Electromagnetic Waves
Satellite communication relies on electromagnetic waves, which are the primary carriers of data over vast distances. These waves travel at the speed of light, bridging gaps between continents, oceans, and even remote regions. They are categorized by their frequency, with higher frequencies enabling faster data transmission but requiring more advanced technology to mitigate challenges like signal loss. Communication satellites predominantly operate within microwave bands, such as C, Ku, and Ka, with each band optimized for specific applications based on its propagation properties and susceptibility to environmental interference.
For instance, the Ka band, known for its high data rates, is commonly used for internet services and video streaming. However, this band is highly susceptible to rain fade, which can weaken the signal during heavy precipitation. Conversely, the C band, though slower, is more robust under adverse weather conditions and is often chosen for television broadcasting in tropical regions where rain is frequent. This careful selection of frequencies ensures optimal performance tailored to each application’s needs.
Signal Transmission Process
To grasp the science behind satellite communication, consider the journey of a signal through its various stages:
- Uplink: The process begins at a ground station equipped with powerful transmitters and antennas. Here, the desired information—be it a video stream, internet data, or voice communication—is encoded into electromagnetic waves. These waves are then directed toward the satellite with precision to ensure minimal signal loss.
- Amplification and Frequency Conversion: Upon reaching the satellite, the signal is received by its onboard antennas and directed to the transponder. The transponder’s primary role is to amplify the signal to restore its strength, as it may have weakened during its travel. Additionally, the signal’s frequency is converted to prevent interference with the original uplink frequency. This step ensures clarity and avoids overlapping with other signals in the network.
- Downlink: The amplified and frequency-modified signal is then transmitted back to Earth. This journey involves ground-based receiving stations or user-end devices, such as satellite dishes installed at homes or businesses. The data is decoded and distributed for use, completing the communication cycle.
For example, during a live international broadcast of a sports event, signals from the event’s location are sent to a satellite via uplink. The satellite processes and relays these signals to multiple ground stations across continents, enabling millions of viewers worldwide to experience the event in near real-time.
Atmospheric Influence and Signal Propagation
The path of electromagnetic waves is not without obstacles. As signals traverse the Earth’s atmosphere, they encounter challenges such as absorption, scattering, and reflection. Higher-frequency signals, like those in the Ka band, are particularly vulnerable to these effects. Rain, snow, and even atmospheric moisture can cause signal degradation, a phenomenon known as rain fade. Engineers address these challenges by implementing robust error-correction algorithms and designing adaptive systems that compensate for environmental disruptions.
Moreover, the curvature of the Earth necessitates careful positioning of satellites to ensure unobstructed signal paths. Ground stations often employ large, highly sensitive antennas capable of receiving weak signals from thousands of kilometers away, further enhancing the reliability of satellite communication systems.
This multi-stage relay of signals, combined with precise engineering, underscores the complexity and ingenuity behind every satellite-enabled communication activity, from everyday internet browsing to critical disaster response coordination.
Orbital Mechanics and Satellite Positioning
Satellites maintain their orbits through a precise balance of gravitational pull and forward velocity, a principle rooted in Newton’s laws of motion. The selection of an orbit—whether geostationary, low Earth orbit, or polar orbit—depends on the satellite’s purpose.
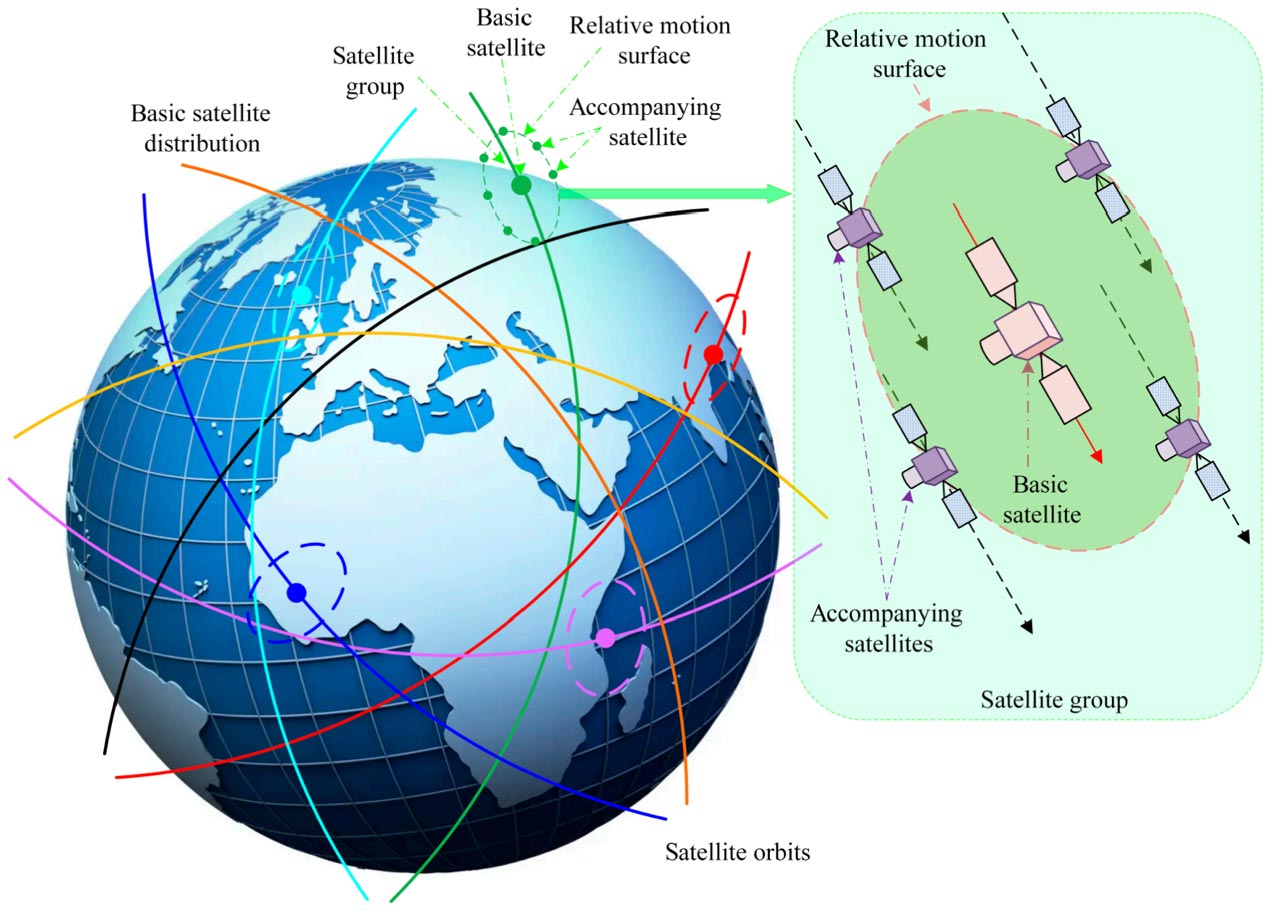
Types of Orbits and Their Applications
- Geostationary Orbits (GEO): These satellites orbit at 35,786 kilometers above the equator, matching the Earth’s rotation to remain stationary relative to the surface. GEO satellites are perfect for continuous broadcasting and long-term communication with fixed locations.
- Low Earth Orbits (LEO): Situated at altitudes between 160 and 2,000 kilometers, LEO satellites move rapidly, completing an orbit in about 90 minutes. Their proximity to Earth minimizes latency, making them ideal for high-speed internet services and real-time data transmission.
- Polar Orbits: These orbits pass over the Earth’s poles, enabling global coverage. They are particularly useful for environmental monitoring and mapping applications, as they provide a comprehensive view of the Earth over time.
Imagine the precision required for a satellite TV system. A GEO satellite must maintain its position accurately to ensure uninterrupted service. Even slight deviations could disrupt the signal, emphasizing the importance of propulsion systems and orbital corrections.
Challenges in Satellite Communication
Operating a satellite communication network is fraught with technical and environmental challenges. Signals must traverse vast distances, and any disruption can significantly affect performance.
Common Challenges
Spectrum Allocation: Limited frequency resources demand careful management to prevent interference between satellites operated by different organizations.
Signal Interference: Atmospheric phenomena like rain, snow, and solar flares can weaken signals, especially in higher-frequency bands such as Ka. Engineers mitigate these effects by designing robust systems and employing error-correction techniques.
Latency: Geostationary satellites introduce delays due to the long travel distance of signals, which can be noticeable during live conversations or gaming. LEO satellites address this issue but require larger constellations for global coverage.
Orbital Debris: The growing amount of space debris poses a collision risk for satellites. Active debris removal and improved tracking technologies are crucial to preserving orbital environments.
Reflection and Discussion
To deepen your understanding of satellite communication, consider the following questions:
- Explore potential advancements in propulsion systems that could enhance satellite longevity and reduce operational costs.
- How do specific frequency bands impact the performance and reliability of satellite systems?
- Why is it critical to maintain precise satellite orbits, and what might happen if corrections are neglected?
Copyright 2024 MAIS Solutions, LLC All Rights Reserved